ref : http://rsync.samba.org/tech_report/
The problem
Imagine you have two files, A and B, and you wish to update B to be the same as A. The obvious method is to copy A ontoB.
Now imagine that the two files are on machines connected by a slow communications link, for example a dial up IP link. IfA is large, copying A onto B will be slow. To make it faster you could compressA before sending it, but that will usually only gain a factor of 2 to 4.
Now assume that A and B are quite similar, perhaps both derived from the same original file. To really speed things up you would need to take advantage of this similarity. A common method is to send just the differences betweenA and B down the link and then use this list of differences to reconstruct the file.
The problem is that the normal methods for creating a set of differences between two files rely on being able to read both files. Thus they require that both files are available beforehand at one end of the link. If they are not both available on the same machine, these algorithms cannot be used (once you had copied the file over, you wouldn't need the differences). This is the problem that rsync addresses.
The rsync algorithm efficiently computes which parts of a source file match some part of an existing destination file. These parts need not be sent across the link; all that is needed is a reference to the part of the destination file. Only parts of the source file which are not matched in this way need to be sent verbatim. The receiver can then construct a copy of the source file using the references to parts of the existing destination file and the verbatim material.
Trivially, the data sent to the receiver can be compressed using any of a range of common compression algorithms, for further speed improvements
The rsync algorithm
Suppose we have two general purpose computers and
. Computer
has access to a fileA and
has access to fileB, where A and B are ``similar''. There is a slow communications link between
and
.
The rsync algorithm consists of the following steps:
-
1.
-
splits the file B into a series of non-overlapping fixed-sized blocks of size S bytes 1. The last block may be shorter than S bytes.
2.
-
For each of these blocks
calculates two checksums: a weak ``rolling'' 32-bit checksum (described below) and a strong 128-bit MD4 checksum.
3.
-
sends these checksums to
.
4.
-
searches through A to find all blocks of length S bytes (at any offset, not just multiples of S) that have the same weak and strong checksum as one of the blocks of B. This can be done in a single pass very quickly using a special property of the rolling checksum described below.
5.
-
sends
a sequence of instructions for constructing a copy of A. Each instruction is either a reference to a block of B, or literal data. Literal data is sent only for those sections of A which did not match any of the blocks of B.
The end result is that gets a copy ofA, but only the pieces of A that are not found in B (plus a small amount of data for checksums and block indexes) are sent over the link. The algorithm also only requires one round trip, which minimises the impact of the link latency.
The most important details of the algorithm are the rolling checksum and the associated multi-alternate search mechanism which allows the all-offsets checksum search to proceed very quickly. These will be discussed in greater detail below.
Rolling checksum
The weak rolling checksum used in the rsync algorithm needs to have the property that it is very cheap to calculate the checksum of a bufferX2 .. Xn+1 given the checksum of bufferX1 .. Xn and the values of the bytesX1 and Xn+1.
The weak checksum algorithm we used in our implementation was inspired by Mark Adler's adler-32 checksum. Our checksum is defined by
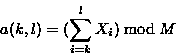
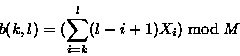
where s(k,l) is the rolling checksum of the bytes . For simplicity and speed, we useM = 216.
The important property of this checksum is that successive values can be computed very efficiently using the recurrence relations


Thus the checksum can be calculated for blocks of length S at all possible offsets within a file in a ``rolling'' fashion, with very little computation at each point.
Despite its simplicity, this checksum was found to be quite adequate as a first level check for a match of two file blocks. We have found in practice that the probability of this checksum matching when the blocks are not equal is quite low. This is important because the much more expensive strong checksum must be calculated for each block where the weak checksum matches.
Checksum searching
Once has received the list of checksums of the blocks ofB, it must search A for any blocks at any offset that match the checksum of some block ofB. The basic strategy is to compute the 32-bit rolling checksum for a block of lengthS starting at each byte of A in turn, and for each checksum, search the list for a match. To do this our implementation uses a simple 3 level searching scheme.
The first level uses a 16-bit hash of the 32-bit rolling checksum and a 216 entry hash table. The list of checksum values (i.e., the checksums from the blocks ofB) is sorted according to the 16-bit hash of the 32-bit rolling checksum. Each entry in the hash table points to the first element of the list for that hash value, or contains a null value if no element of the list has that hash value.
At each offset in the file the 32-bit rolling checksum and its 16-bit hash are calculated. If the hash table entry for that hash value is not a null value, the second level check is invoked.
The second level check involves scanning the sorted checksum list starting with the entry pointed to by the hash table entry, looking for an entry whose 32-bit rolling checksum matches the current value. The scan terminates when it reaches an entry whose 16-bit hash differs. If this search finds a match, the third level check is invoked.
The third level check involves calculating the strong checksum for the current offset in the file and comparing it with the strong checksum value in the current list entry. If the two strong checksums match, we assume that we have found a block ofA which matches a block of B. In fact the blocks could be different, but the probability of this is microscopic, and in practice this is a reasonable assumption.
When a match is found, sends
the data inA between the current file offset and the end of the previous match, followed by the index of the block inB that matched. This data is sent immediately a match is found, which allows us to overlap the communication with further computation.
If no match is found at a given offset in the file, the rolling checksum is updated to the next offset and the search proceeds. If a match is found, the search is restarted at the end of the matched block. This strategy saves a considerable amount of computation for the common case where the two files are nearly identical. In addition, it would be a simple matter to encode the block indexes as runs, for the common case where a portion ofA matches a series of blocks of B in order.
Pipelining
The above sections describe the process for constructing a copy of one file on a remote system. If we have a several files to copy, we can gain a considerable latency advantage by pipelining the process.
This involves initiating two independent processes. One of the processes generates and sends the checksums to
while the other receives the difference information from
and reconstructs the files.
If the communications link is buffered then these two processes can proceed independently and the link should be kept fully utilised in both directions for most of the time.
Results
To test the algorithm, tar files were created of the Linux kernel sources for two versions of the kernel. The two kernel versions were 1.99.10 and 2.0.0. These tar files are approximately 24MB in size and are separated by 5 released patch levels.
Out of the 2441 files in the 1.99.10 release, 291 files had changed in the 2.0.0 release, 19 files had been removed and 25 files had been added.
A ``diff'' of the two tar files using the standard GNU diff utility produced over 32 thousand lines of output totalling 2.1 MB.
The following table shows the results for rsync between the two files with a varying block size.2
block | matches | tag | false | data | written | read |
size | hits | alarms | ||||
300 | 64247 | 3817434 | 948 | 5312200 | 5629158 | 1632284 |
500 | 46989 | 620013 | 64 | 1091900 | 1283906 | 979384 |
700 | 33255 | 571970 | 22 | 1307800 | 1444346 | 699564 |
900 | 25686 | 525058 | 24 | 1469500 | 1575438 | 544124 |
1100 | 20848 | 496844 | 21 | 1654500 | 1740838 | 445204 |
In each case, the CPU time taken was less than the time it takes to run ``diff'' on the two files.3
The columns in the table are as follows:
-
block size
- The size in bytes of the checksummed blocks. matches
- The number of times a block of B was found in A. tag hits
- The number of times the 16 bit hash of the rolling checksum matched a hash of one of the checksums from B. false alarms
- The number of times the 32 bit rolling checksum matched but the strong checksum didn't. data
- The amount of file data transferred verbatim, in bytes. written
-
The total number of bytes written by
including protocol overheads. This is almost all file data.
read
-
The total number of bytes read by
including protocol overheads. This is almost all checksum information.
The results demonstrate that for block sizes above 300 bytes, only a small fraction (around 5%) of the file was transferred. The amount transferred was also considerably less than the size of the diff file that would have been transferred if the diff/patch method of updating a remote file was used.
The checksums themselves took up a considerable amount of space, although much less than the size of the data transferred in each case. Each pair of checksums consumes 20 bytes: 4 bytes for the rolling checksum plus 16 bytes for the 128-bit MD4 checksum.
The number of false alarms was less than 1/1000 of the number of true matches, indicating that the 32 bit rolling checksum is quite good at screening out false matches.
The number of tag hits indicates that the second level of the checksum search algorithm was invoked about once every 50 characters. This is quite high because the total number of blocks in the file is a large fraction of the size of the tag hash table. For smaller files we would expect the tag hit rate to be much closer to the number of matches. For extremely large files, we should probably increase the size of the hash table.
The next table shows similar results for a much smaller set of files. In this case the files were not packed into a tar file first. Rather, rsync was invoked with an option to recursively descend the directory tree. The files used were from two source releases of another software package called Samba. The total source code size is 1.7 MB and the diff between the two releases is 4155 lines long totalling 120 kB.
Availability
An implementation of rsync which provides a convenient interface similar to the common UNIX command rcp has been written and is available for download fromftp://rsync.samba.org/pub/rsync.
About this document ...
The rsync algorithm
This document was generated using the LaTeX2HTML translator Version 98.1p1 release (March 2nd, 1998)
Copyright © 1993, 1994, 1995, 1996, 1997, Nikos Drakos, Computer Based Learning Unit, University of Leeds.
The command line arguments were:
latex2html tech_report.tex.
The translation was initiated by Andrew Tridgell on 1998-11-09