首先这个系列的第一个单元是空中机器人,博客如下:
1 Robotics: Aerial Robotics 第1+2周 课程学习记录及课后习题解答
1 Robotics: Aerial Robotics 第3+4周 课程学习记录及课后习题解答
2 Robotics: Computational Motion Planning 第1周(内含Dijkstra 和 A* MATLAB代码手把手教学)课后习题解答
2 Robotics: Computational Motion Planning 第2+3+4周 课后习题解答
此课程在Coursera需要科学上网才能观看,但是b站有人搬运,只是无中英字幕,放一下B站和Coursera的课程链接
- UP主 博主自己做的字幕版本 这个单元… 只有英文(持续更新)
- Coursera的链接介绍
此文仅为听课记录以及做题思考,有所错误的地方或是好的想法欢迎评论区交流。(点个赞就更好了,鼓励一下~)
- 2.25:听说评论说这个单元… 没有编程作业,做作业像阅读理解一样,emm怎么的也得更完…
- 2.27:这一节节的视频也太长了,划分句子,打轴真的累死了,烦请看视频的各位b站来个三连吧…
WEEK 1
1.1.1 Why and how do animals move
1.In Newton’s
F
=
m
a
F=ma
F=ma what does
F
F
F denote?
Force
2.Why is bioinspiration encouraged over biomimicry
All of the above
3.If an animal or robot wants to turn left, it should swing its tail
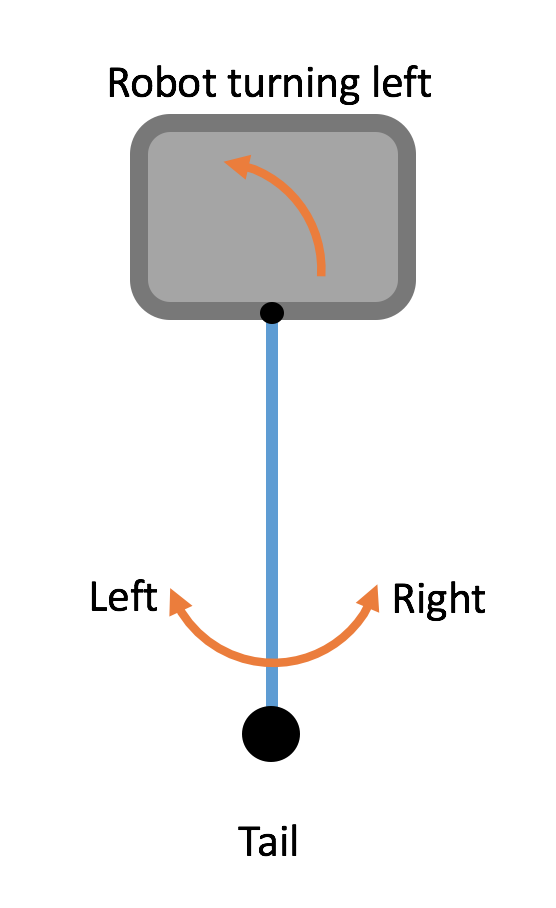
4.Which is an example of intelligence “baked into” the body?
Kangaroo tendons acting as springs
Quiz 1.1.2 Bioinspiration
1.The speed of airplanes is often described in units of Mach (the speed of sound at standard sea level conditions). What is the speed of Helios 2 in Mach?
207
2.Acceleration is sometimes measured in g (acceleration due to gravity at the Earth’s surface). What is the cheetah’s acceleration in units of g?
1.3
解释:记得看清这里的单位是,几个g的加速度
3.Turning left “at the house with the red roof” is an example of navigation using which part of the hippocampus?
Place cells
4.Turning left “after 25 meters” is an example of navigation using which part of the hippocampus?
Grid cells
1.1.3 Legged Mobility: dynamic motion and the management of energy
1.In the above image, we see three robots with the leg forces on the ground drawn in blue. Match the robots with their resulting jumps.
Robot “b” will jump the highest up, robot “a” will jump the furthest sideways.
2.Hamiltonian systems have their total energy (sum of potential and kinetic energies) conserved. Which of these can best be modeled as a Hamiltonian system.
A pendulum in a clock swinging back and forth
3.Assuming these two motors are about the same size, which one will likely produce more torque and which one will have higher inertia?
The motor on the right will produce more torque and have higher inertia
4.Which are examples of programming work?
A robot opening a door
A robot running over rough terrain
WEEK 2
2.1.1 Walking like a rimless wheel
1.Energetic cost of transport is a measure commonly used to evaluate the energetic efficiency of robotic locomotion, and is given by the energy needed to travel a given distance with a robot, divided by the energy needed to lift the robot the same distance vertically against gravity. Which of the below trigonometric relations gives the energetic cost of transport (COT) for the rimless wheel powered by gravity as a function of ramp angle? Use the horizontal distance traveled in your calculations to represent distance traveled.
COT=Tan(rampAngle)
2.The Cornell Ranger has a mass of 9.91 kg and walked a total distance of 65,243 m using 1,774,800 J of battery energy. What is the cost of transport for the Cornell Ranger? Assume a gravitational acceleration of 9.81 m/s^2 in calculating your answer.
0.28
解释:COT = E / mgd; E = Energy input of the system, m = mass, d = distance, g = standard gravity
COT = 1774800/(9.919.8165243) = 0.28
3.What ramp angle is required to give a cost of transport of 0.2 for the rimless wheel, which is approximately equal to the efficiency of human walking?
11 degrees
解释:arctan(0.2)=11
4.We’ve seen that energy is often used very efficiently for movement in biology as compared to in robots. The cost of storing energy (in terms of weight) is another factor that comes into play in determining locomotion ability – the more weight is stored in fuel, the more mass the actuators need to move! Let’s compare how much it weights to store a given amount of energy in biological fuel – i.e. food – as compared with electricity to power a robot.
How many kg of battery weight would it be necessary for a human-like robot to walk a marathon? Assume that a marathon takes 5960 KJ to walk (this is an extremely roughly estimate assuming a 72 kg person walking it with a energetic cost of transport of 0.2), and that the lithium polymer batteries have a mass of 0.002kg per KJ of energy storage. Round to the nearest kilogram.
Just to put this in perspective, this same energy can be contained in less than 7 candy bars, which have a total weight of less than 0.4kg!
12
解释:0.002*5960=11.96 四舍五入 12
2.1.2 Running like a spring-loaded pendulum
1.Given a robot with a leg length of 0.4m, what is the estimated maximum possible walking speed in meters per second according to the Froude number? Assume an acceleration due to gravity of 10m/s^2.
2
解释:F=v^2/(gl),v=sqrt(1100.4)=2
2.Assume that the energetically optimal walk-to-run transition speed for a robotic biped occurs at a Froude number of 0.5. What is the expression for the optimal speed of transitioning from walking to running in terms of leg length d? Assume a gravitational acceleration of 10m/s^2. Enter you answer in simplest form, using sqrt() to represent any square roots. (Ex: The square root of 2d is written as sqrt(2d)).
sqrt(0.510d)
3.Which of the following energy plots best represents the energy during stance of the center-of-mass of a robot running with SLIP-like dynamics? The blue curve shows kinetic energy and the red curve shows gravitational potential energy. Assume the horizontal axis is time and the vertical axis is energy.
如此图
4.Here is actual data for the center-of-mass trajectory of a robot running primarily in the directions labeled y and z. From this data, which template best explains the motion observed?
LLS
2.1.3 Controlling the spring-loaded inverted pendulum
1.Download the MATLAB file called Quiz_2_1_1_Raibert_Hopper. You run the simulation by running Quiz_2_1_1_Question_1 with no arguments.
Your first task is to tune the thrust duration using the slider so that the hopper reaches a steady state hopping height between 1m and 1.1m (denoted by the horizontal green and red lines in the generated plot) at the end of the simulation. Give the resulting thrust duration in seconds that meets these goals as your answer.
0.00071429
2.Now we will tune the forward speed controller. You run the simulation by running Quiz_2_1_1_Question_2 with no arguments.
Recall that the neutral point is estimated at each step, and that the forward speed controller consists of a proportional controller that puts the toe in front of or behind the neutral point to obtain the desired speed. What proportional gain to the forward speed controller causes the robot to accelerate from rest to a speed between 0.9 m/s and 1.1 m/s without ever going over 1.1 m/s?
0.087439
3.Now we will tune the pitch controller to achieve a desired pitch of π 12 \frac{\pi}{12} 12π radians. You run the simulation by running Quiz_2_1_1_Question_3 with no arguments.
Your task is to finish writing the PD controller contained in pitchController.m. Keep a derivative term of kd_phi = 5. What value of kp_phi bounds the pitch within the green and red lines in the graph generated by the simulation?
5
代码如下:
function Tphi = pitchController(phi,phiDesired,dphi_dt)
kd_phi = 5;
kp_phi = 5;
Tphi= kp_phi*(phiDesired-phi)+kd_phi*(0-dphi_dt);
4.Now we will put all 3 controller parts together. You run the simulation by running Quiz_2_1_1_Question_4 with no arguments.
In this simulation you combine the 3 previous controllers. Can you make the robot move how you want it to? The intention of this question is just for you to gain some intuition regarding how these controllers interact, and to have some fun seeing that very simple controllers can get the robot to move robustly. When you are done, simply enter 42 as the answer below.
42
这个题是随意题… 就是结合上面的后,自己可以控制玩一玩
2.2.1 Metrics and Scaling: mass, length, strength
1.If a cube’s side length is doubled, what happens to its perimeter, surface area, and volume?
Permeter:2x
Area: 4x
Voolume: 8x
2.A small ice cube with side length L and a larger ice cube of length 2L are left sitting out in the sun. If the small ice cube takes 10 minutes to melt, how long will the large one take, in minutes?
Hint: You don’t need to know anything about thermodynamics to solve this; heat transfer (the rate at which the ice cube heats up) is proportional to the surface area of the ice cube, and thermal mass (the amount of heat required to melt the ice cube) is proportional to volume. We assume that the melting time is proportional to this ratio.
20
Solution: 20 -> 10 minutes * Volume / Area
这里有个英文文档解释
3.If a larger bone in a larger animal is 3x longer than a smaller bone in a smaller animal, and compressive stress in both bones is the same, how many times thicker must the larger bone be?
Hint: stress is mass divided by area. Assuming constant density of the animals, mass is proportional to volume. Also assume that the volume scales with length^3. Remember that the primary compression occurs axially along the length of the bone. How much larger must the thickness be to maintain the same (mass)/(cross sectional area) ratio?
Correct answers should be submitted with one decimal place, (e.g, 23.5)
0.11
V = 333 = 27 -> sqrt(V) = 5.19 ~ 5.2
这里有个英文文档解释
4.Pair the following scenarios with the dominant mode of loading:
- a rubber band being stretched
- a diving board being jumped on
- a can being stood on
- a bolt being tightened
1) tensio 2) bending 3) compression 4) torsion
2.2.2 Materials, manufacturing, and assembly
1.With everything else being equal (stiffness, strength, dimensions, etc.) which type of material will heat up more if stressed in tension until failure?
Ductile
2.If a 10cm diameter, 0.5m long cylinder is loaded with 20kg mass in tension, what is the stress (force divided by cross-sectional area) in the material in Pascals (the SI unit for stress: N/m^2)? (Remember, we are concerned with axial tension loads)
24972.4
解释:stress=F/A;F=mg,A=pi*r^2
3.If the above cylinder then stretches by 8cm, what is the strain (extension normalized by initial length) in %?
16
解释:0.08/0.5=16%
4.Necking is a phenomena where plastic deformation induces significant strain resulting in decreased cross sectional area. If the above cylinder experiences “necking” will the stress in the neck be larger or smaller than the stress in the rest of the sample?
Lager
2.2.3 Design: figures of merit, robustness
1.Question 1
Given the following material properties:
Aluminum 6061-T6 has a density of 2.7 g/cm^3, a Young’s modulus of 69 GPa, and an ultimate tensile strength of 310 MPa.
ABS Plastic has a density of 1.3 g/cm^3, a Young’s modulus of 2.2 GPa, and an ultimate tensile strength of 70 MPa.
If a “spring” were to be made by pulling a 10cm long cylinder of material in tension with a desired stiffness of 5000kN/m, what should the diameter (in millimeters) be for an Aluminum “spring”?
This wikipedia page may be helpful, specifically the section : “Force exerted by stretched or contracted material”.
3.04
解释:
F
L
0
=
E
A
Δ
L
FL_0=E A \Delta L
FL0=EAΔL,A是底面积,F力,E杨氏模量
2.If the “spring” were to be made of ABS plastic instead, what should the diameter be (in millimeters)?
17.01
3.What would be the mass of the aluminum “spring” (in grams)?
1.96
解释:质量=密度*体积
4.What would be the mass of the plastic “spring” (in grams)?
31.97
5.If the aluminum “spring” were pulled 3mm, would it break?
Yes
6.If the plastic “spring” were pulled 3mm, would it break?
No
2.3.1 Actuator technologies
1.Which of these tasks is significantly dependent on high actuator bandwidth (frequency at which actuator can work well)?
High speed pick and place
A legged robot catching itself after a stumble
2.The speed torque curve establishes a relationship between these two physical properties in electric motors. Since power is the product of torque and angular speed, where does maximum power occur?
At 1/2 no-load speed
3.Torque in an electromagnetic actuator is proportional to current by the constant
K
t
K_t
Kt ( in Nm/A, often specified by motor manufacturers). The dominant mode of wasted energy in these actuators is due to Joule heating which scales with current squared. Where does maximum wasted energy occur?
At stall (no speed)
4.In the above diagram, if both arrows are held fixed and the position of the central node is
x
x
x and its velocity is
v
v
v, what is the differential equation representing this system? Assume the spring has rest length
x
0
x_0
x0.
F
+
b
v
=
k
(
x
−
x
0
)
F+bv=k(x-x_0)
F+bv=k(x−x0)
WEEK 3
3.1.1 Review of kinematics (MATLAB)
1.For all of this quiz, download and unzip the attached file.
Using MATLAB, open the Q1 folder and edit “computeForwardKinematics.m” and compute forward kinematics for a single revolute link, replacing the trivial solution (x=0, y=0). Then run "singlelinkforward.m"To check that this is correct, drag the slider to
θ
1
=
2
\theta_1 = 2
θ1=2what is the x coordinate of the end effector?
Assume
L
1
=
1
L_1=1
L1=1.
-0.41
2.Using MATLAB, open the Q2 folder and edit “computeRRForwardKinematics.m” and compute forward kinematics for a revolute-revolute robot, replacing the trivial solution for both the elbow (joint connecting
L
1
L_1
L1 and
L
2
L_2
L2 ) and end effector (orange dot). To check that this is correct, run “rrForward.m” and drag the sliders to
θ
1
=
1
\theta_1 = 1
θ1=1 and
θ
2
=
0.75
\theta_2 = 0.75
θ2=0.75what is the y coordinate of the end effector?
Assume
L
1
=
1
L_1=1
L1=1, and
L
2
=
1
L_2=1
L2=1.
1.83
3.Using MATLAB, open the Q3 folder and edit “computeRRInverseKinematics.m” and compute inverse kinematics for a revolute-revolute robot. To check that this is correct, run “rrInverse.m” and drag the sliders to
x
=
0
x = 0
x=0 and
y
=
1.5
y = 1.5
y=1.5. What is the value of
θ
2
\theta_2
θ2, assuming that
0
<
θ
1
<
π
2
0<\theta_1 <\frac{\pi}{2}
0<θ1<2π and
0
<
θ
2
<
2
π
0<\theta_2 <2\pi
0<θ2<2π?
Assume
L
1
=
1
L_1=1
L1=1, and
L
2
=
1
L_2=1
L2=1.
Note: There are technically two correct configurations, yet we are limiting the possible angles of
θ
1
\theta_1
θ1 in such a way that only one of the solutions is valid.
1.44
解释:其实道理,我觉得高中学了的人都能懂… 以下仅作为参考
PS:这个需要在2019b的MATLAB下运行,不然会报错 未定义函数或变量 ‘eqnproblem’。
X=0;Y=1.5;%题目要求的值
theta = optimvar('theta',2);
ls1 = fcn2optimexpr(@(theta)cos(theta(1)+theta(2))+cos(theta(2)),theta);
eq1 = ls1 == X;
ls2 = fcn2optimexpr(@(theta)sin(theta(1)+theta(2))+sin(theta(2)),theta);
eq2 = ls2 == Y;
prob = eqnproblem;
prob.Equations.eq1 = eq1;
prob.Equations.eq2 = eq2;
show(prob);
x0.theta = [0.001 0.0001];%此处是因为题目进行了大于0的约束,所以从0.0001开始
[sol,fval,exitflag] = solve(prob,x0);
rads1=sol.theta(1);
rads2=sol.theta(2);
disp(sol.theta)
4.Using MATLAB, open the Q4 folder and edit “computeMiniForwardKinematics.m” and compute the forward kinematics for a parallel robot. To check that this is correct, run “miniForwardNoToe.m” and drag the sliders to
θ
1
=
3.5
\theta_1 = 3.5
θ1=3.5 and
θ
2
=
1.5
\theta_2 = 1.5
θ2=1.5 what is the value of x?
Assume the shorter link is of length 1 and the longer link is of length 2.
1.02
3.1.2 Introduction to dynamics and control
1.One possible simplification to the dynamics of the spring loaded inverted pendulum (SLIP) model is to neglect gravity altogether. Using your knowledge of Lagrangian mechanics, which of the below equations of motion are generated from this simplification?
Note: The kinetic and potential energies are given using polar coordinates. The correct answer below is in simplest form and involves a cancellation. You can assume that the radial coordinate never goes to zero.
m
r
¨
−
k
(
r
0
−
r
)
−
m
r
θ
˙
2
=
0
r
θ
¨
+
2
r
˙
θ
˙
=
0
\begin{array}{l} m\ddot r - k({r_0} - r) - mr{{\dot \theta }^2} = 0\\ r\ddot \theta + 2\dot r\dot \theta = 0 \end{array}
mr¨−k(r0−r)−mrθ˙2=0rθ¨+2r˙θ˙=0
2.Which of the following PD controllers – used to directly give motor commands u – is implemented correctly for the goal of having the motors to track the desired state? Let the desired state is given by the subscript “des”, the proportional term be given by kp, and the derivative term be given by kd.
u
=
k
p
(
θ
d
e
s
−
θ
)
+
k
d
(
θ
˙
d
e
s
−
θ
˙
)
u = {k_p}({\theta _{des}} - \theta ) + {k_d}({\dot \theta _{des}} - \dot \theta )
u=kp(θdes−θ)+kd(θ˙des−θ˙)
3.PD control allows the motors to add stiffness (via the P term) and damping (via the D term) in different directions. Often when controlling robotic legs it proves useful to have these directions align with some coordinate system at the foot. For example, you might want the motors to make the leg seem very stiff when the foot pushes against the ground in the vertical direction but not as much in the horizontal direction.
Assume a 2 degree of freedom leg actuated by 2 motors. Which of the following equations is a PD controller that gives motor commands to track a desired x and y foot position such that the leg stiffness and damping can be independently controlled in the x and y directions? Hint: it may be helpful to review the end of the kinematics section showing how forces at the end effector are mapped to motor torques.
Let
J
J
J be the kinematic Jacobian, mapping motor velocities into toe velocities. Let
k
p
x
k_{px}
kpx,
k
p
y
k_{py}
kpy be the
x
x
x and
y
y
y proportional gains, and
k
d
x
k_{dx}
kdx,
k
d
y
k_{dy}
kdy be the
x
x
x and
y
y
y derivative gains.
u
=
J
(
k
p
x
(
x
d
e
s
−
x
)
+
k
d
x
(
x
˙
d
e
s
−
x
˙
)
k
p
y
(
y
d
e
s
−
y
)
+
k
d
y
(
y
˙
d
e
s
−
y
˙
)
)
u = J\left( {\begin{array}{cc} {{k_{px}}({x_{des}} - x) + {k_{dx}}({{\dot x}_{des}} - \dot x)}\\ {{k_{py}}({y_{des}} - y) + {k_{dy}}({{\dot y}_{des}} - \dot y)} \end{array}} \right)
u=J(kpx(xdes−x)+kdx(x˙des−x˙)kpy(ydes−y)+kdy(y˙des−y˙))
3.2.1 Sprawled posture runners
1.Assume the center of mass (CoM) is at (0,0)(0,0), the left corner is at (-1,0), the bottom is at (1,-1), and the top is at (1,1). What is
d
1
d1
d1? Enter 3 decimal places.
0.447
解释:大家在草稿纸上画图,然后同面积底x高… emm具体是1/根号5
2.The lecture introduced the idea of a support polygon (see the figure below and [Ting, 1994] for more information). The cockroach is “quasistatically stable” when the projection of the CoM is within the support polygon at all times. In this question and the next, we will think about the limits this model imposes on locomotion speed.
Assume that the CoM moves along a vertical line through the origin as the cockroach runs forward, and that the toe locations are fixed at (-1,0), (1,-1), and (1,1), in units of cm (about right for a small cockroach).
What is the maximum CoM displacement that keeps a positive quasistatic stability margin (in cm)?
1
解释:这个地方就是如果三个点不动,蟑螂往前能移多少,也就是两边与y的交点长度,… 大家画一下三角形在坐标轴的图就知道我在说些啥了
3.(building off the previous question)
Assume that the CoM moves along a vertical line through the origin as the cockroach runs forward, and that the toe locations are fixed at (-1,0), (1,-1), and (1,1), in units of cm (about right for a small cockroach).
Assuming the stride frequency and duty cycle are such that each tripod is maintained for 0.5 seconds, what is the maximum speed such that quasistatic stability is guaranteed (in cm/s)?
2
解释:如果能移动1cm,而时间是0.5s,所以最大是2cm/s
4.In this question you will think about if RHex’s “C” legs are better or worse than stick legs for a vertical leap. Imagine the two leg designs on a vertical rail and frictionless floor (as shown above); the legs rotate in the direction of the red arrow and the body is propelled upwards.
Recall Gavin’s lecture on effective mechanical advantage (EMA) from 3.1.1. Which of these curves roughly captures the EMA versus height trend for each leg?
Hint: you don’t need to calculate the kinematics. Think about how much the hip joint rotates per infinitesimal displacement in height of the “robot” as a function of the height. Start with the stick leg.
green - half circle leg, blue - stick leg
1.First watch this gif (you can also save it to your computer or watch here if it isn’t playing) of my foster kitten performing a gait transition.
Trot to gallop
解释:这几个都好好看视频就知道了
2.This ostrich is a:
Digitigrade
解释:鸵鸟是啥种类的动物…
3.From the evidence of this cost of transport (CoT) plot of animals and robots from MIT, which of these is a more efficient mode of locomotion?
Swimming
4.From the evidence of this CoT plot of animals and robots from MIT, how much power is a human running at 3m/s consuming (in Watts)?
743
解释:CoT=metalbolic power/(mgv),也就是
P
o
w
e
r
=
80
×
9.8
×
3
×
1
0
−
0.5
Power=80\times 9.8\times 3\times 10^{-0.5}
Power=80×9.8×3×10−0.5
3.2.3 Bipeds
1.You’ve seen the SLIP model a few times now. You may have noticed that this model does not allow for a biped to stand still (recall inverted pendulums from 1.2.3). What additional actuation/appendage is required for a monoped (sagittal projection of a biped) to stably stand? (There may be more than one correct answer, and assume that multiple answers are independent of each other, i.e., A OR B and not A and B)
Ankle torque
An actuated tail attached at the hip (but not allowed to touch the ground)
A tail allowed to touch the ground
2.How many degrees of freedom (DOFs) does sagittal Jerboa (model above) have in stance (toe is pinned to the ground)?
Image from De and Koditschek [2015b]
Hint: First, count the total unconstrained DOFs for the Jerboa, including
(
x
,
z
)
(x, z)
(x,z), the leg DOFs
(
θ
1
,
θ
2
)
(\theta_1, \theta_2)
(θ1,θ2), and the orientation DOFs
(
ϕ
1
,
ϕ
2
)
(ϕ_1,ϕ_2)
(ϕ1,ϕ2). In stance, you have to consider the TWO constraints imposed by the fact that the toe is pinned to the ground.
4
3.How many non-degenerate* degrees of freedom (DOFs) does sagittal Jerboa (model above) have in flight (massless leg)?
*We call a DOF with zero apparent inertia (such as a massless end effector on an actuated joint) degenerate, since theoretically any actuator torque would cause infinite acceleration.
Image from De and Koditschek [2015b]
Hint: start from the total DOFs in the unconstrained Jerboa (as in the previous question), but now eliminate the DOFs that have zero mass associated with them.
4
Simply stabilized SLIP (MATLAB)
1.In the following cartoon robots, an encoder measures leg angle relative to body, and an IMU measures absolute body angle.
In this cartoon robot, assuming the control input is desired leg angle, which sensors need to be used to implement the simply stabilized controller? (Keep in mind the ‘disk body’ has no degree of freedom)
None
2.In this cartoon robot, which sensors are needed to implement the Raibert stepping controller (from week 2)?
Encoder
3.In this cartoon robot, assuming the control input is desired leg angle (relative to the body), which sensors are needed to implement the simply stabilized controller?
IMU
4.In this cartoon robot, assuming the control input is desired leg angle (relative to the body), which sensors are needed to implement the Raibert stepping controller?
IMU
Encoder
5.In the attached zip file, you will find a MATLAB GUI (run “slipHW.m”). In the GUI that appears, you must set the parameters through the input boxes (as described below), and click “run” to run the simulation and present results.
You will find a simplistic implementation of SLIP with a tunable fixed stepping angle. You will change the value of the stepping angle (in radians) to get a sense of the basin of this simple controller.
What is a valid stepping angle (in units of radians) for speed 0.5 m/s?
Hint: change the stepping angle till there are sustained bounces, i.e., the stride-to-stride apex z z z and speed x ˙ \dot x x˙ should not change much. A “close enough” answer is sufficient.
Hint 2: the correct answer lies between 0.12 and 0.14 radians, and you may need to go down to
±
0.0001
\pm 0.0001
±0.0001 radians of precision to get it to work. This question is meant to convey how small the basin of the fixed stepping angle controller really is!
0.1294
6.Experiment with a couple of more values of speed, and finding a good stepping angle. Which of the following plot trends represents the stepping angle – speed relation well? NOTE: Enter “gray”, “green”, “blue”, or “red” (not including quotes).
blue
WEEK 4
4.1.1 Sequential and Parallel Composition
1.In the execution of this sequential composition, how many controllers need to be sequenced for initial point
x
1
x_1
x1? (enter a numeric answer)
2
2.In this scenario, Φ1 makes the goal an attracting fixed point. Which of these is a correct reason that controllers Φ2 and Φ3 are needed in addition?
The basin of
Φ
1
Φ_1
Φ1 is not large engough
3.Why is
Φ
3
→
Φ
1
Φ_3→Φ_1
Φ3→Φ1 not a valid sequential composition?
The basin of
Φ
1
Φ_1
Φ1 does not include the goal of
Φ
3
Φ_3
Φ3
4.2.1 Why is parallel hard?
1.Which of these is a template for a trotting horse?
Hint: this is a review of earlier material, specifically around 1:30–2:30 of lecture 2.1.1 where Jeff introduces templates
SLIP
2.Recall from lecture the de-coupled RL-RC circuit ( R 21 = ∞ ) (R_{21} = \infty) (R21=∞), where x ˙ = A x \dot x=Ax x˙=Ax, and A A A is a 2x2 diagonal matrix with values A 11 A_{11} A11 and $A_{22}. What is the solution x 1 ( t ) x_1(t) x1(t) if starting at t = 0 t=0 t=0? Use “x10” for x 1 ( 0 ) x_1(0) x1(0), “x20” for x 2 ( 0 ) x_2(0) x2(0), and “A11” for A 11 A_{11} A11 etc. To denote e x e^x ex, use “exp(x)”.
Hint: for those in need of a refresher on ODEs, you might find this helpful.
x10exp(A11t)
3.Which of these is the reason the ”heavy toe” simulation of the Raibert hopper fails?
The stepping controller affects the pitch
4.2.3a RHex
1.Why was RHex designed to have one actuator per hip?
To have adequate robot specific power (power/mass)
2.Which are some of the steps that had to be taken to anchor a pogo stick on RHex (referred to as “tuning” in the lecture)?
The compliance (stiffness magnitudes) had to be tuned
The clock had to be tuned properly
4.3.1 Compositions of vertical hoppers
1.How many virtual legs are used in a quadrupedal walk? (refer: 3.2.2)
2.How many virtual legs are used by a hopping kangaroo?
3.Suppose the cartoon robot below is in stance (toe is pinned to the ground), and a hip torque is applied in the direction of the arrow. Then
4.Assume all the virtual or physical toes are in stance (i.e. pinned to the ground). The arrows in the left figure are physical leg extension forces, and the arrow in the right figure is a virtual hip torque. Note that this counterclockwise virtual hip torque would result in a clockwise acceleration of the body.
To apply the shown virtual hip torque on the right, in the physical model on the left,
5.Assume the physical robot on the left is in stance. The equivalent “virtual leg” is shown in the image on the right. In this question we think about how physical torques can move the virtual hip. Assume that a torque in the direction of the arrows is positive.
Imagine the right physical hip applies 0 Nm. Then, a torque of 1 Nm applied by the left physical hip moves the virtual hip to the left. Similarly, if the left hip applies -1 Nm and the right hip applies 0 Nm, the virtual hip moves to the right.
Which of the following would translate the pivot point of the virtual hip to the left?
MATLAB: composition of vertical hoppers
1.We have made a very simplistic MATLAB simulation with a Minitaur-like body and a composition of identical, independent vertical hoppers. This means that in the simulation, each leg is controlled as if it were a vertical hopper, and the controllers are truly decoupled.
The following questions should help you see the power of this kind of composition, and also how the body design might affect behavior.
u X
2.Select d=0.2d=0.2 and leave it for the following three questions.
Increasing the gain from 0, with d=0.2d=0.2, find the smallest value of vertical hopper gain such that both of the vertical hoppers have sustained bounces.
Hint: look at the hybrid mode plot; “3” means that both legs are in stance. Make sure that the system doesn’t settle in to mode 3 after a few bounces; i.e., modes other than 3 (when either vertical hopper has some aerial phase) are visible till the end of the simulation.
12
3.With d=0.2d=0.2, find a value of VH gain such that “bounding” occurs, i.e. the vertical hoppers get out of sync, and there is no double stance (i.e. the hybrid mode does not reach 3 after a few initial bounces)
30 X
4.With d=0.2d=0.2, what is the value of VH gain such that the equilibrium zz (of the CoM) is \approx 0.2≈0.2.
36
5.Now we shall see how the body dynamics (specifically, as parameterized by the length of the body) affects our composition. Change to d=0.1d=0.1 and leave it for the following three questions.
With d=0.1d=0.1, find a value of vertical hopper gain such that ”bounding” occurs, i.e. the vertical hoppers get out of sync, and there is no double stance (i.e. the hybrid mode does not reach 3 after a few initial bounces).
Note: You will observe that the shorter body does not like to passively desynchronize the vertical hoppers as well as the longer body…telling us that more active desynchronization is needed (not implemented in this simulation).
15 X
6.With d=0.1d=0.1, what is the value of vertical hopper gain such that the equilibrium zz (of the CoM) is \approx 0.2≈0.2?
Note: it might oscillate about this value unless you run the simulation for a longer time, but try to guess the steady-state value it is converging to.
40
4.3.2 Same composition, different bodies
4.3.3 Same body, different compositions
1.The inertial reorientation template plant (pictured below) dynamically only has 1 DOF, because
of angular momentum conservation
2.Suppose we modify the template plant for inertial reorientation and pin the body away from the CoM. Gravity is present. How many DOFs does this system have?
2
4.3.4 Transitions
1.To get some idea of the ground reaction complex (GRC), take the example of an nn-legged stool being dropped to the ground, and assume that as it falls, contacts can be made but never broken (i.e. if a leg touches the ground it doesn’t lift off again), and also that there are no “simultaneous” contacts. Define the “sitting” mode as the one where all legs are in contact.
Define the term “word” as a sequence of contact modes in a transitional behavior. For example, 000→001→011→111 is a single word comprised of 4 letters (contact modes). For further reference into the vocabulary analogy take a look at
https://repository.upenn.edu/ese_papers/662/
How many possible “words” are there from aerial to sitting for n=2n=2?
2
2.(Same setup as previous question) How many words are there from aerial to sitting for n=3n=3?
6
3.The three digits inside the robot are a binary string representing current contact mode, with 1 signifying an active contact, and 0 and inactive one. If “LB = left body, RB = right body, T = toe”, the correct sequence of labels is
LB,T,RB
4.First watch this leap (you can also save the gif to your computer)
Assuming the contact labels are in the sequence {nose, leg, rear, tail}, which of these is the correct completion of the leap word corresponding to the video clip?
1010→1011→1001→1101→0101→
Hint: think about which contacts are the last to break?
0001→0000