提示:文章写完后,目录可以自动生成,如何生成可参考右边的帮助文档
文章目录
Preface
Our models are developed mainly for signals in the Ultra High Frequency (UHF) and Super High Frequency (SHF) bands, from 0.3 − 3 GHz 0.3-3 ~\text{GHz} 0.3−3 GHz and 3 − 30 GHz 3-30~ \text{GHz} 3−30 GHz, respectively.
All transmitted and received signals we consider are real. This is due to modulators and demodulators are built using oscillators that can generate real sinusoids only ( not complex exponentials ). Thus, the transmitted signal output from a modulator is a real signal. Similarly, the demodulator only extracts the real part of the signal at its input.
Transmitted Signal Model
We model the transmitted signal as
s
(
t
)
=
ℜ
{
u
(
t
)
e
j
(
2
π
f
c
t
+
ϕ
0
)
}
=
ℜ
{
u
(
t
)
}
cos
(
2
π
f
c
t
+
ϕ
0
)
−
ℑ
{
u
(
t
)
}
sin
(
2
π
f
c
t
+
ϕ
0
)
=
x
(
t
)
cos
(
2
π
f
c
t
+
ϕ
0
)
−
y
(
t
)
sin
(
2
π
f
c
t
+
ϕ
0
)
\begin{aligned} s(t) &=\Re\left\{u(t) e^{j\left(2 \pi f_{c} t+\phi_{0}\right)}\right\} \\ &=\Re\{u(t)\} \cos \left(2 \pi f_{c} t+\phi_{0}\right)-\Im\{u(t)\} \sin \left(2 \pi f_{c} t+\phi_{0}\right) \\ &=x(t) \cos \left(2 \pi f_{c} t+\phi_{0}\right)-y(t) \sin \left(2 \pi f_{c} t+\phi_{0}\right) \end{aligned}
s(t)=ℜ{u(t)ej(2πfct+ϕ0)}=ℜ{u(t)}cos(2πfct+ϕ0)−ℑ{u(t)}sin(2πfct+ϕ0)=x(t)cos(2πfct+ϕ0)−y(t)sin(2πfct+ϕ0) where
u
(
t
)
=
x
(
t
)
+
j
y
(
t
)
u(t) = x(t) + jy(t)
u(t)=x(t)+jy(t) is a complex baseband signal with in-phase component
x
(
t
)
=
R
{
u
(
t
)
}
x(t) = \mathcal{R}\{u(t)\}
x(t)=R{u(t)}, quadrature component
y
(
t
)
=
ℑ
{
u
(
t
)
}
y(t)=\Im\{u(t)\}
y(t)=ℑ{u(t)}, bandwidth
B
B
B, and power
P
u
P_u
Pu. The signal
u
(
t
)
u(t)
u(t) is called the complex envelope or complex lowpass equivalent signal of
s
(
t
)
s(t)
s(t). This is due to the magnitude of
u
(
t
)
u(t)
u(t) is that of
s
(
t
)
s(t)
s(t), and the phase of
u
(
t
)
u(t)
u(t) is that of
s
(
t
)
s(t)
s(t) relative to the carrier frequency
f
c
f_c
fc and the initial phase offset
ϕ
0
\phi_0
ϕ0. This is a common representation for bandpass signals with
B
<
<
f
c
B << f_c
B<<fc, as it allows signal manipulation via
u
(
t
)
u(t)
u(t) irrespective of the carrier frequency and phase. The power in the transmitted signal
s
(
t
)
s(t)
s(t) is
P
t
=
P
u
/
2
P_t=P_u / 2
Pt=Pu/2
Received Signal Model
The received signal will have similar form: r ( t ) = ℜ { v ( t ) e j ( 2 π f c t + ϕ 0 ) } , r(t) = \Re \{ v(t) e^{j(2 \pi f_c t + \phi_0 )} \}, r(t)=ℜ{v(t)ej(2πfct+ϕ0)}, where the complex baseband signal is v ( t ) v(t) v(t) will depend on the channel through which s ( t ) s(t) s(t) propagates. In particular, if s ( t ) s(t) s(t) is transmitted through a time-invariant channel then v ( t ) = u ( t ) ∗ c ( t ) v(t) = u(t) * c(t) v(t)=u(t)∗c(t), where c ( t ) c(t) c(t) is the equivalent lowpass channel impulse response for the channel .
Doppler shift
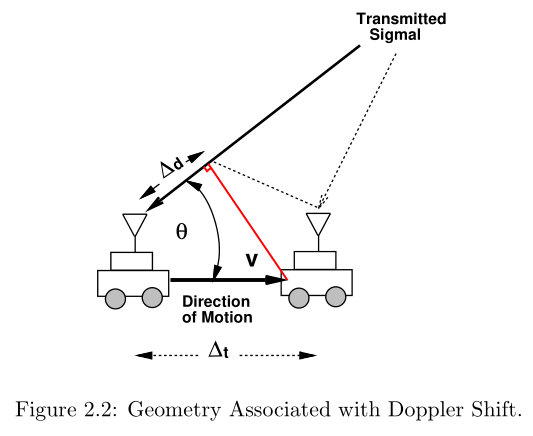
The received signal may have a Doppler shift of
f
D
=
v
cos
θ
/
λ
f_D = v \cos \theta / \lambda
fD=vcosθ/λ associated with it, where
θ
\theta
θ is the arrival angle of the received signal relative to the direction of motion,
v
v
v is the receiver velocity towards the transmitter in the direction of motion, and
λ
=
c
/
f
c
\lambda = c / f_c
λ=c/fc is the signal wavelength (
c
c
c is the the speed of light). The geometry associated with the Doppler shift is shown in Fig. 2.2. The Doppler shift results from the fact that transmitter or receiver movement over a short time interval
△
t
\triangle t
△t causes a slight change in distance
△
d
=
v
△
t
cos
θ
\triangle d = v \triangle t \cos \theta
△d=v△tcosθ that the transmitted signal needs to travel to the receiver. The phase change due to this path length difference is
∆
φ
=
2
π
v
∆
t
c
o
s
θ
/
λ
∆ φ = 2πv∆tcosθ/λ
∆φ=2πv∆tcosθ/λ. The Doppler frequency is then obtained from the relationship between signal frequency and phase:
f
D
=
1
2
π
Δ
ϕ
Δ
t
=
v
cos
θ
/
λ
.
f_{D}=\frac{1}{2 \pi} \frac{\Delta \phi}{\Delta t}=v \cos \theta / \lambda.
fD=2π1ΔtΔϕ=vcosθ/λ. If the receiver is moving towards the transmitter, i.e.
−
π
/
2
≤
θ
≤
π
/
2
− \pi /2 ≤ θ ≤ \pi /2
−π/2≤θ≤π/2, then the Doppler frequency is positive, otherwise it is negative.
Here, we ignore the Doppler term in the free-space and ray tracing models of this chapter, since for typical urban vehicle speeds (
60
mph
60~ \text{mph}
60 mph) and frequency (around
1
GHz
1 ~ \text{GHz}
1 GHz), it is less than
70
Hz
70~ \text{Hz}
70 Hz.
Path Loss
Suppose s ( t ) s(t) s(t) of power P t P_t Pt is transmitted through a given channel, with corresponding received signal r ( t ) r(t) r(t) of power P r P_r Pr, where P r P_r Pr is averaged over any random variations due to shadowing. We define the linear path loss of the channel as the ratio of transmit power to receive power: P L (dB) = 10 log 10 P t P r . P_L \text{(dB)} = 10\log_{10} \frac{P_t}{P_r}. PL(dB)=10log10PrPt.
In general, path loss is a nonnegative number since the channel does not contain active elements, and thus can only attenuate the signal. The path gain in dB \text{dB} dB is defined as the negative of the path loss: P G = − P L = 10 log 10 ( P r / P t ) , P_G = -P_L = 10\log_{10} (P_r / P_t), PG=−PL=10log10(Pr/Pt), which is generally a negative number. With shadowing, the received power will include the effects of path loss and an additional random component due to blockage from objectives.
Received Signal of Free-Space Path
Consider a signal transmitted through free space to a receiver located at distance d from the transmitter. Assume there are no obstructions between the transmitter and receiver. The channel model associated with this transmission is called a Line-Of-Sight (LOS) channel, and the corresponding received signal is called the LOS signal or ray. Free-space path loss introduces a complex scale factor, resulting in the received signal:
r
(
t
)
=
ℜ
{
λ
G
l
e
−
j
(
2
π
d
/
λ
)
4
π
d
u
(
t
)
e
j
2
π
f
c
t
}
,
r(t)=\Re\left\{\frac{\lambda \sqrt{G_{l}} e^{-j(2 \pi d / \lambda)}}{4 \pi d} u(t) e^{j 2 \pi f_{c} t}\right\},
r(t)=ℜ{4πdλGle−j(2πd/λ)u(t)ej2πfct}, where
G
l
\sqrt{G_l}
Gl is the product of the transmit and receive antenna Field Radiation Patterns (FRP, 场辐射图) in the LOS direction, and the phase shift
e
−
j
(
2
π
d
/
λ
)
e^{-j(2\pi d / \lambda)}
e−j(2πd/λ) (
e
−
j
(
2
π
f
c
τ
e^{-j(2 \pi f_c \tau}
e−j(2πfcτ, where delay
τ
=
d
/
c
~\tau = d/c
τ=d/c) is due to the distance
d
d
d the wave travels.
Free-Space Path Loss
The power in the transmitted signal s ( t ) s(t) s(t) is P t P_t Pt, so the ratio of received to transmitted power computed by is P r P t = [ G l λ 4 π d ] 2 . \frac{P_r}{P_t} = \left[ \frac{ \sqrt{G_l } \lambda }{4 \pi d} \right]^2. PtPr=[4πdGlλ]2. Thus, the received signal power falls off inversely proportional to the square of the distance d d d between the transmit and receive antennas. The received signal power is also proportional to the square of the signal wavelength, so as the carrier frequency increases, the received power decreases. This dependence of received power on the signal wavelength λ \lambda λ is due to the effective area of the receive antenna. However, the antenna gain of highly directional antennas can increase with frequency, so the receive power may actually increase with frequency for highly directional links.
The received power can be expressed in dBm \text{dBm} dBm as P r ( dBm ) = P t ( dBm ) + 10 log 10 ( G l ) + 20 l o g 10 ( λ ) − 20 log 10 ( 4 π ) − 20 log 10 ( d ) . P_r ~(\text{dBm}) = P_t ~(\text{dBm}) + 10 \log_{10} ~(G_l) + 20 log_{10} (\lambda) - 20\log_{10}(4 \pi) - 20\log_{10}(d) . Pr (dBm)=Pt (dBm)+10log10 (Gl)+20log10(λ)−20log10(4π)−20log10(d).
Free-space path loss is defined as the path loss of the free-space model: P L ( dB ) = 10 log 10 P t P r = − 10 log 10 G l λ 2 ( 4 π d ) 2 . P_L ~ (\text{dB}) = 10 \log_{10} \frac{P_t}{P_r} = -10 \log_{10} \frac{ {G_l } \lambda^2 }{ (4 \pi d)^2}. PL (dB)=10log10PrPt=−10log10(4πd)2Glλ2.
The free-space path gain is thus P G = − P L = 10 log 10 G l λ 2 ( 4 π d ) 2 . P_G =- P_L = 10 \log_{10} \frac{ {G_l } \lambda^2 }{ (4 \pi d)^2}. PG=−PL=10log10(4πd)2Glλ2.